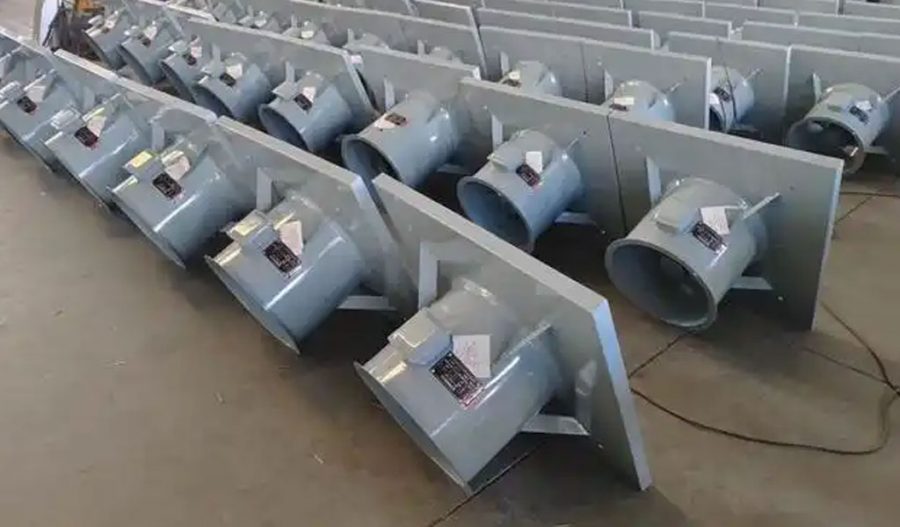
In the domains of real estate and architecture, the term “ventilation shell” is not a standard or widely recognized phrase within the conventional lexicon of building design or property development. However, for the purposes of this article, we interpret “ventilation shell” as a conceptual framework referring to the structural and systemic components of a building designed to facilitate ventilation, encompassing the building envelope, mechanical systems, and architectural features that collectively ensure adequate air exchange and quality. This interpretation aligns with the principles of indoor air quality management, energy efficiency, and occupant comfort, which are central to modern architectural practice. The ventilation shell, in this context, can be understood as the integrated assembly of elements that govern airflow, including walls, windows, ducts, fans, and other purpose-built openings, tailored to the building’s intended use and environmental conditions.
Ventilation, broadly defined, is the intentional or unintentional movement of air into, out of, or within a building to maintain a healthy and comfortable indoor environment. The ventilation shell, therefore, serves as the physical and functional interface between the indoor space and the external environment, balancing the need for fresh air with energy conservation and structural integrity. This article provides a comprehensive exploration of the ventilation shell, examining its components, types, design considerations, performance metrics, and its role in various building typologies. By delving into the technical, environmental, and regulatory aspects, we aim to offer a thorough understanding of how ventilation shells contribute to sustainable and occupant-centric building design.
Historical Context of Ventilation in Architecture
The concept of ventilation has been integral to architecture since antiquity, with early civilizations employing passive design strategies to manage airflow. Ancient Mesopotamian and Egyptian structures, for instance, incorporated courtyards and windcatchers to channel breezes through living spaces, leveraging natural forces like wind and thermal buoyancy. The Roman hypocaust system, used for heating, also facilitated air circulation by drawing fresh air through underfloor channels. These early practices laid the groundwork for understanding the ventilation shell as a system reliant on the building’s form and orientation.
In medieval Europe, ventilation was often rudimentary, with open windows and chimneys serving as primary air exchange mechanisms. However, the lack of systematic design led to poor indoor air quality, particularly in densely populated urban areas. The Renaissance period saw advancements in architectural theory, with figures like Leon Battista Alberti emphasizing the importance of air movement for health. By the 18th and 19th centuries, the Industrial Revolution spurred innovations in mechanical ventilation, driven by the need to ventilate factories, hospitals, and public buildings.
Industrial Era and Mechanical Ventilation
The advent of mechanical ventilation systems in the 19th century marked a significant evolution in the ventilation shell. Pioneers like David Boswell Reid designed ventilation systems for the British Houses of Parliament, using steam-powered fans to draw air through dedicated ducts. This period also saw the development of negative pressure ventilators, such as the iron lung, which, while primarily medical, underscored the growing understanding of controlled air movement. The ventilation shell during this era began to incorporate mechanical components, such as fans and ductwork, alongside traditional passive elements like windows and vents.
The 20th century brought further refinements, with the widespread adoption of heating, ventilation, and air-conditioning (HVAC) systems. The ventilation shell became more complex, integrating advanced materials, sensors, and controls to optimize airflow. The energy crises of the 1970s highlighted the need for energy-efficient ventilation, prompting research into hybrid systems that combine natural and mechanical methods. Today, the ventilation shell is a critical component of sustainable architecture, shaped by advances in materials science, computational modeling, and regulatory frameworks.
Defining the Ventilation Shell
Conceptual Framework
The ventilation shell can be defined as the totality of a building’s structural and mechanical elements designed to facilitate air exchange, maintain indoor air quality, and regulate thermal comfort. It encompasses the building envelope (walls, roofs, floors, and openings), ventilation systems (natural, mechanical, or hybrid), and ancillary components like filters, dampers, and sensors. Unlike the broader concept of the building envelope, which focuses on thermal and moisture barriers, the ventilation shell is specifically concerned with airflow dynamics and pollutant management.
The ventilation shell operates as a dynamic system, responding to internal and external conditions such as occupant density, outdoor air quality, and climatic factors. Its design must balance competing priorities, including energy efficiency, occupant health, and structural durability. For instance, a tightly sealed ventilation shell may reduce energy loss but increase the risk of indoor pollutant accumulation, necessitating mechanical ventilation. Conversely, a highly permeable shell may enhance natural ventilation but compromise thermal performance.
Components of the Ventilation Shell
The ventilation shell comprises several key components, each contributing to its overall functionality:
- Building Envelope: The physical barrier between indoor and outdoor environments, including walls, roofs, windows, and doors. The envelope’s permeability, insulation, and opening design significantly influence ventilation performance.
- Openings: Purpose-built apertures such as windows, vents, louvers, and trickle ventilators that allow controlled air exchange. Adjustable openings enable occupants to modulate airflow based on need.
- Mechanical Systems: Fans, ducts, and air-handling units (AHUs) that drive forced ventilation. These systems may include supply fans, exhaust fans, or balanced configurations.
- Natural Ventilation Features: Architectural elements like wind towers, solar chimneys, and atria that leverage passive forces to move air.
- Filtration and Treatment: Filters, ultraviolet germicidal irradiation (UVGI) systems, and other devices that remove pollutants and pathogens from the airstream.
- Controls and Sensors: Automated systems that monitor air quality, temperature, and humidity, adjusting ventilation rates accordingly.
These components interact to form a cohesive ventilation shell, tailored to the building’s typology, climate, and occupancy patterns.
Types of Ventilation Shells
Natural Ventilation Shells
Natural ventilation shells rely on passive forces—wind pressure and thermal buoyancy—to drive airflow through the building. They are characterized by a permeable building envelope with strategically placed openings, such as windows, vents, and skylights. Natural ventilation is most effective in climates with moderate temperatures and consistent wind patterns, where outdoor air quality is sufficient to meet indoor needs.
Key features of natural ventilation shells include:
- Cross Ventilation: Airflow driven by pressure differences between windward and leeward sides of the building, facilitated by opposing windows or vents.
- Stack Effect: Vertical airflow induced by temperature differences, where warmer indoor air rises and exits through high openings, drawing cooler air in from below.
- Windcatchers and Solar Chimneys: Traditional and modern elements that enhance airflow by capturing wind or amplifying thermal gradients.
Natural ventilation shells are energy-efficient but depend heavily on climate, building orientation, and occupant behavior. In urban environments, noise and pollution may limit their feasibility, necessitating hybrid or mechanical solutions.
Mechanical Ventilation Shells
Mechanical ventilation shells employ fans and ductwork to force air movement, offering precise control over ventilation rates and air quality. They are essential in buildings with high occupant densities, sensitive equipment, or poor outdoor air quality. Mechanical systems can be categorized as follows:
- Supply Ventilation: Fans push outdoor air into the building, creating positive pressure that forces stale air out through leaks or vents. Common in residential and commercial spaces.
- Exhaust Ventilation: Fans extract indoor air, creating negative pressure that draws fresh air in through passive vents. Often used in kitchens, bathrooms, and industrial settings.
- Balanced Ventilation: Combines supply and exhaust fans to maintain neutral pressure, ensuring equal air exchange. Heat recovery ventilators (HRVs) and energy recovery ventilators (ERVs) enhance efficiency by transferring heat and moisture between airstreams.
Mechanical ventilation shells are highly adaptable but require significant energy and maintenance. Their design must account for duct leakage, fan efficiency, and filter performance to optimize performance.
Hybrid Ventilation Shells
Hybrid ventilation shells integrate natural and mechanical systems, switching between modes based on environmental conditions or occupant needs. For example, a building may rely on natural ventilation during mild weather but activate mechanical systems during extreme temperatures or high pollution levels. Hybrid shells offer flexibility and energy savings but require sophisticated controls to manage transitions effectively.
Infiltration and Adventitious Ventilation
Infiltration refers to the uncontrolled entry of outdoor air through leaks in the building envelope, such as gaps around windows or poorly sealed joints. While not a deliberate component of the ventilation shell, infiltration can significantly impact air exchange rates. Adventitious ventilation, a related concept, occurs when vents or openings designed for ventilation are influenced by unintended pressures, such as strong winds. Both phenomena must be considered in ventilation shell design to ensure consistent performance.
Design Considerations for Ventilation Shells
Climatic Influences
The design of a ventilation shell is heavily influenced by local climate, which dictates the balance between natural and mechanical ventilation. In temperate climates, natural ventilation shells with large, operable windows may suffice, while hot, humid climates require mechanical systems to control moisture and prevent condensation. Cold climates demand tightly sealed envelopes to minimize heat loss, often paired with balanced ventilation systems like HRVs.
Wind patterns, temperature gradients, and humidity levels shape the placement and size of openings, as well as the selection of mechanical components. For instance, coastal regions with consistent breezes may prioritize cross ventilation, while arid regions may use evaporative cooling integrated into the ventilation shell.
Building Typology and Occupancy
The intended use of a building—residential, commercial, industrial, or institutional—determines the ventilation shell’s complexity. High-occupancy spaces like schools and offices require robust mechanical systems to handle carbon dioxide and pollutant loads, while residential buildings may rely on simpler natural or exhaust systems. Industrial facilities, with their unique emission profiles, demand specialized ventilation shells to capture and remove hazardous fumes.
Occupant behavior also plays a role. In residential settings, occupants may open windows to supplement mechanical ventilation, while in commercial buildings, automated systems often dominate due to centralized control. The ventilation shell must accommodate these patterns while maintaining performance.
Energy Efficiency and Sustainability
Energy efficiency is a critical consideration in ventilation shell design, given that ventilation accounts for a significant portion of a building’s energy consumption. Strategies to enhance efficiency include:
- Heat Recovery: HRVs and ERVs transfer heat between incoming and outgoing air, reducing heating and cooling demands.
- Demand-Controlled Ventilation (DCV): Sensors adjust ventilation rates based on occupancy or pollutant levels, minimizing energy waste.
- High-Efficiency Fans: Variable-speed fans optimize airflow while reducing power consumption.
- Passive Design: Optimizing building orientation, window placement, and thermal mass to enhance natural ventilation.
Sustainable materials, such as low-emission insulation and recyclable ductwork, further enhance the ventilation shell’s environmental performance.
Indoor Air Quality and Health
The primary function of the ventilation shell is to maintain indoor air quality (IAQ) by diluting and removing pollutants, including carbon dioxide, volatile organic compounds (VOCs), and particulate matter. Poor IAQ is linked to health issues like respiratory disorders, allergies, and cognitive impairment. The ventilation shell must therefore incorporate effective filtration, regular maintenance, and monitoring to ensure clean air delivery.
In the context of infectious diseases, such as COVID-19, ventilation shells play a critical role in reducing airborne transmission. High ventilation rates, combined with UVGI or HEPA filtration, can significantly lower viral concentrations in indoor spaces.
Regulatory and Standards Compliance
Ventilation shell design is governed by a range of standards and codes, including:
- ASHRAE Standards 62.1 and 62.2: Specify minimum ventilation rates for commercial and residential buildings, respectively.
- International Mechanical Code (IMC): Outlines requirements for mechanical ventilation systems.
- United Kingdom Building Regulations Part F: Mandates ventilation provisions for new and renovated buildings.
- OSHA Guidelines: Address ventilation in occupational settings to control workplace hazards.
Compliance ensures that the ventilation shell meets safety, health, and energy performance benchmarks, while also addressing local climate and building use requirements.
Performance Metrics and Evaluation
Ventilation Rate
The ventilation rate, measured in liters per second (L/s) or cubic feet per minute (CFM), quantifies the volume of outdoor air delivered to a space. It is a key indicator of the ventilation shell’s effectiveness. ASHRAE Standard 62.1 recommends ventilation rates based on occupancy and space type, typically ranging from 5–20 CFM per person. Higher rates are required in spaces with elevated pollutant loads, such as laboratories or kitchens.
Air Changes per Hour (ACH)
Air changes per hour (ACH) measure how often the air in a space is replaced by fresh air. For example, an ACH of 6 indicates that the entire volume of air is replaced six times per hour. ACH is particularly relevant in healthcare and industrial settings, where high ventilation rates are needed to control contaminants.
Ventilation Effectiveness
Ventilation effectiveness describes how well a ventilation shell delivers fresh air to occupied zones and removes pollutants. It is influenced by airflow patterns, diffuser placement, and mixing conditions. A well-designed ventilation shell achieves high effectiveness by ensuring uniform air distribution and minimizing short-circuiting (where fresh air exits without mixing).
Energy Performance
The energy performance of a ventilation shell is evaluated based on its power consumption, heat recovery efficiency, and impact on overall building energy use. Metrics like specific fan power (SFP), measured in watts per liter per second (W/L/s), quantify fan efficiency. Energy-efficient ventilation shells typically achieve SFPs below 0.5 W/L/s.
Indoor Air Quality Metrics
IAQ is assessed through metrics like carbon dioxide concentration (typically maintained below 1000 ppm), particulate matter (PM2.5 and PM10), and VOC levels. Sensors and air quality monitors provide real-time data to evaluate the ventilation shell’s performance in maintaining a healthy indoor environment.
Comparative Analysis of Ventilation Shell Types
To illustrate the differences between natural, mechanical, and hybrid ventilation shells, the following table provides a detailed comparison across key parameters:
Parameter | Natural Ventilation Shell | Mechanical Ventilation Shell | Hybrid Ventilation Shell |
---|---|---|---|
Primary Mechanism | Passive forces (wind, thermal buoyancy) | Fans and ductwork | Combination of passive and mechanical systems |
Energy Consumption | Low (no mechanical components) | High (fan and system operation) | Moderate (varies by mode) |
Control Precision | Low (dependent on weather and occupant behavior) | High (automated controls and sensors) | Moderate to high (automated switching between modes) |
Climate Suitability | Best in temperate climates with good outdoor air quality | Suitable for all climates, including extreme conditions | Adaptable to varying climates |
Initial Cost | Low (minimal equipment) | High (fans, ducts, AHUs) | Moderate to high (combined systems) |
Maintenance | Low (cleaning of vents and windows) | High (filter replacement, fan servicing, duct cleaning) | Moderate (maintenance of both passive and mechanical components) |
Indoor Air Quality | Variable (dependent on outdoor air quality and airflow patterns) | High (with proper filtration and maintenance) | High (combines benefits of both systems) |
Applications | Residential, small commercial, traditional buildings | High-occupancy buildings, hospitals, laboratories | Schools, offices, mixed-use buildings |
Examples | Traditional courtyard houses, windcatcher-equipped buildings | HVAC-equipped skyscrapers, industrial facilities | Green buildings with automated windows and supplemental HVAC |
Challenges | Limited control, noise, and pollution infiltration | Energy costs, duct leakage, maintenance complexity | System integration, control complexity |
This table highlights the trade-offs between ventilation shell types, guiding designers in selecting the most appropriate system for a given project.
Case Studies of Ventilation Shells in Practice
Residential Buildings
In residential architecture, ventilation shells often prioritize simplicity and cost-effectiveness. A notable example is the BedZED (Beddington Zero Energy Development) project in London, UK, which employs a hybrid ventilation shell. The development features wind-driven ventilation cowls that channel fresh air into homes, supplemented by mechanical ventilation with heat recovery during colder months. The ventilation shell’s design reduces energy consumption by 50% compared to conventional homes while maintaining excellent IAQ.
Commercial Buildings
The Bullitt Center in Seattle, USA, dubbed the “greenest commercial building in the world,” showcases an advanced natural ventilation shell. Operable windows, automated louvers, and a carefully designed atrium facilitate cross and stack ventilation, eliminating the need for mechanical cooling in the mild Pacific Northwest climate. The ventilation shell’s performance is enhanced by real-time IAQ monitoring, ensuring occupant comfort and energy efficiency.
Industrial Facilities
Industrial ventilation shells are tailored to manage high pollutant loads. The Tesla Gigafactory in Nevada, USA, employs a mechanical ventilation shell with extensive ductwork, high-capacity exhaust fans, and HEPA filtration to control fumes and particulates from battery production. The system’s balanced configuration minimizes energy loss while protecting workers from hazardous exposures.
Healthcare Facilities
Hospitals require sophisticated ventilation shells to prevent nosocomial infections. The Khoo Teck Puat Hospital in Singapore integrates natural and mechanical ventilation, using courtyards and operable windows for patient rooms and high-efficiency HVAC systems for operating theaters. The ventilation shell’s design reduces airborne pathogen transmission while promoting patient recovery through access to fresh air.
Technological Innovations in Ventilation Shells
Smart Ventilation Systems
Advances in sensor technology and artificial intelligence have transformed ventilation shell design. Smart ventilation systems use real-time data on CO2 levels, temperature, and occupancy to adjust airflow dynamically. For example, the Nest thermostat integrates with ventilation systems to optimize energy use, while platforms like Aircuity monitor multiple IAQ parameters to ensure compliance with standards.
Advanced Materials
Innovative materials enhance the performance of ventilation shells. Phase-change materials (PCMs) in walls and ceilings regulate temperature, reducing the need for mechanical ventilation. Nanoporous membranes in vents improve filtration efficiency, capturing ultrafine particles without restricting airflow. Self-cleaning coatings on ducts and filters reduce maintenance costs and extend system lifespan.
Building Information Modeling (BIM)
BIM enables architects and engineers to simulate ventilation shell performance during the design phase. By modeling airflow, heat transfer, and pollutant dispersion, BIM optimizes the placement of openings, ducts, and fans, ensuring efficient and effective ventilation. This technology is particularly valuable for complex projects like hospitals and data centers.
Renewable Energy Integration
Ventilation shells increasingly incorporate renewable energy sources to reduce carbon footprints. Solar-powered fans, for instance, drive mechanical ventilation in off-grid buildings, while geothermal heat pumps precondition incoming air, enhancing energy efficiency. These innovations align with global sustainability goals, such as the Paris Agreement’s targets for net-zero emissions.
Challenges and Limitations
Energy Trade-Offs
While ventilation shells are essential for IAQ, they can contribute significantly to energy consumption, particularly in mechanical systems. Balancing ventilation rates with energy efficiency remains a challenge, especially in extreme climates where heating or cooling demands are high. Innovations like DCV and ERVs mitigate this issue but require upfront investment.
Maintenance and Durability
Mechanical ventilation shells demand regular maintenance to prevent performance degradation. Clogged filters, leaky ducts, and worn-out fans can compromise IAQ and increase energy costs. Natural ventilation shells, while less maintenance-intensive, are susceptible to weathering and require periodic cleaning to ensure functionality.
Outdoor Air Quality
In urban areas with poor outdoor air quality, ventilation shells must incorporate advanced filtration to protect occupants from pollutants like PM2.5, ozone, and nitrogen oxides. This adds complexity and cost, particularly for natural ventilation systems that rely on unfiltered outdoor air.
Occupant Behavior
The effectiveness of ventilation shells, especially natural ones, depends on occupant behavior. Inconsistent window operation or improper use of mechanical controls can undermine performance. Education and automation are critical to ensuring optimal use of the ventilation shell.
Future Directions
Net-Zero and Passive House Standards
The rise of net-zero and Passive House standards is reshaping ventilation shell design. These frameworks prioritize ultra-low energy consumption, requiring highly efficient ventilation systems with advanced heat recovery and airtight envelopes. Future ventilation shells will likely integrate seamlessly with renewable energy systems, achieving carbon neutrality without compromising IAQ.
Biophilic Design
Biophilic design, which emphasizes connections to nature, is influencing ventilation shell aesthetics and functionality. Green walls, living roofs, and indoor plants can enhance air quality and thermal comfort, complementing traditional ventilation components. These features align with growing demand for wellness-focused architecture.
Climate Adaptation
As climate change intensifies, ventilation shells must adapt to shifting conditions, such as increased heatwaves, humidity, and air pollution. Resilient designs will incorporate flexible systems capable of switching between natural and mechanical modes, as well as robust filtration to address worsening outdoor air quality.
Policy and Incentives
Governments worldwide are introducing policies to promote energy-efficient ventilation. Incentives for green building certifications, such as LEED and BREEAM, encourage the adoption of advanced ventilation shells. Future regulations may mandate real-time IAQ monitoring and stricter ventilation standards, driving innovation in the field.
Conclusion
The ventilation shell, as conceptualized in this article, represents a critical nexus of architecture, engineering, and environmental science. By integrating the building envelope, ventilation systems, and advanced technologies, it ensures healthy, comfortable, and sustainable indoor environments. From ancient windcatchers to modern smart HVAC systems, the evolution of the ventilation shell reflects humanity’s ongoing quest to balance occupant well-being with resource efficiency.
As buildings become more airtight and energy-conscious, the ventilation shell’s role in managing IAQ and energy performance will only grow. Innovations in materials, controls, and renewable energy integration promise to enhance its capabilities, while challenges like climate change and urban pollution demand adaptive solutions. By understanding and optimizing the ventilation shell, architects, engineers, and policymakers can create buildings that not only shelter but also nurture their occupants, paving the way for a healthier and more sustainable future.
Maximize Tooling and CNC Metal Spinning Capabilities.
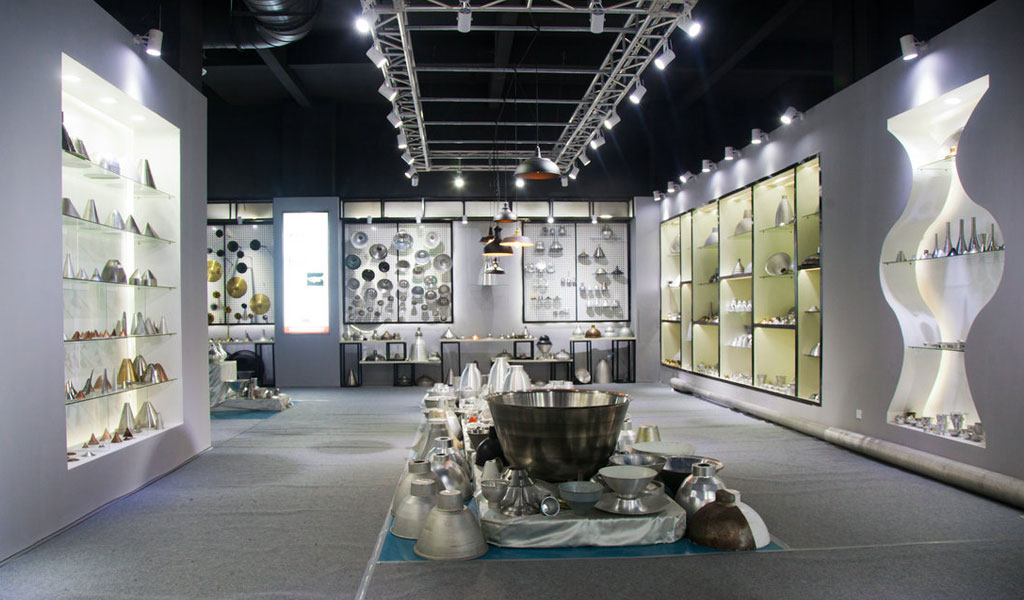
At BE-CU China Metal Spinning company, we make the most of our equipment while monitoring signs of excess wear and stress. In addition, we look into newer, modern equipment and invest in those that can support or increase our manufacturing capabilities. Our team is very mindful of our machines and tools, so we also routinely maintain them to ensure they don’t negatively impact your part’s quality and productivity.
Talk to us today about making a rapid prototype with our CNC metal spinning service. Get a direct quote by chatting with us here or request a free project review.
BE-CU China CNC Metal Spinning service include : CNC Metal Spinning,Metal Spinning Die,Laser Cutting, Tank Heads Spinning,Metal Hemispheres Spinning,Metal Cones Spinning,Metal Dish-Shaped Spinning,Metal Trumpet Spinning,Metal Venturi Spinning,Aluminum Spinning Products,Stainless Steel Spinning Products,Copper Spinning Products,Brass Spinning Products,Steel Spinning Product,Metal Spinnin LED Reflector,Metal Spinning Pressure Vessel,